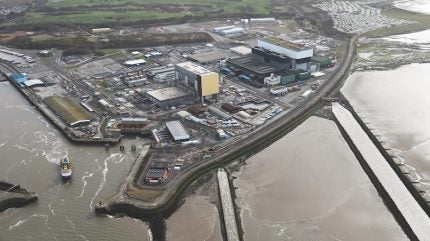
A new report by the Dalton Nuclear Institute, part of the UK’s University of Manchester, has explored how renewables and nuclear power can jointly contribute to achieving net zero.
Focused on the UK, the report ‘The road to net zero: renewables and nuclear working together’, nonetheless applies to many nations and regions. It states that while renewable energy is expected to play a large role in achieving net zero ambitions, their variability opens the door to alternatives, including nuclear. The report argues that to achieve net zero greenhouse gas emissions by 2050 there will need to be a significant increase in the proportion of energy that is delivered by electricity, and which must be predominantly low carbon. This is reflected in the anticipated shift to a greater role for electricity to support transport, domestic heating and industrial processes, for example.
To meet this demand the bulk of 2050 electricity supply is anticipated to be from renewable generation, largely wind and solar. However, these resources are unavoidably variable, so additional support is required to managing the inherent intermittencies and deliver a functioning electricity grid.
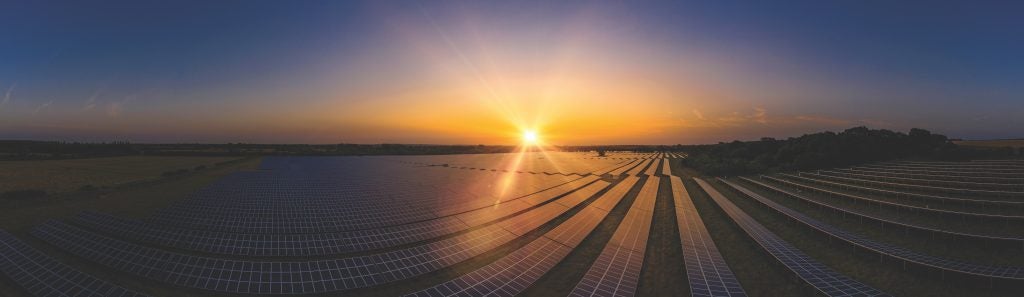
Options to cope with the variability of renewables include natural gas, with or without carbon capture, as well as storage capacity. Shorter-term storage could be met by batteries, medium-term storage by pumped hydropower and in the longer-term storage of hydrogen for use in gas turbines. Another option, the report states, is the increased deployment of nuclear energy.
Alternatives to flexible gas
The Dalton analysis notes that many of the low-carbon electricity generation and grid system scenarios examined to date have included the use of unabated gas generation, but operating for only a small percentage of the time. This “low capacity factor operation‚ means that all the capital and operating costs must be recovered by selling a small amount of electricity. This means that the unit production cost of the electricity will be very high. With a low capacity factor even seemingly cheap sources of electricity become expensive.
However, rather than using gas which is currently deployed to deliver system flexibility, the report argues that another possible solution to the conundrum of filling in the gaps left by variable renewables is nuclear. Without resorting to the construction of large, dedicated power plants to be run at very low capacity factors during low renewables output the Dalton report argues nuclear power could be used for both grid support and cogeneration applications, with most of the output directed at the cogeneration applications.
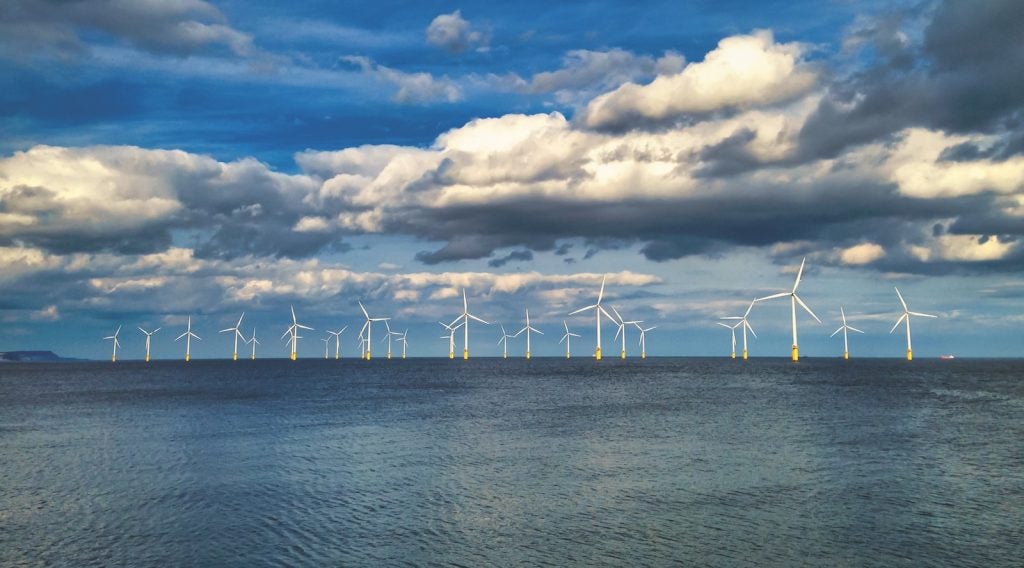
The key challenge is that the capacity factor of nuclear energy must be kept high in order to keep costs low, but flexibility is also needed to supply electricity when demand is high and renewable supply is low.
As planned renewable capacities are expected to meet maximum demand, the supporting capacities must also be similarly sized. If net zero is to be achieved, sources of low-carbon energy besides electricity must be delivered for roles which are currently provided by fossil fuels given that electricity makes up only around a fifth of national energy consumption in the UK, for example.
Flexible nuclear
For a number of technical reasons, the light water reactors (LWRs) typically employed for nuclear power generation today have limitations on the flexibility of generation that can be achieved. They are also limited by the fact that lower fractions of time spent on generation mean lower capacity factors and lead to rapidly increasing generation costs.
However, the report notes that nuclear energy can supply both heat and electricity in a “cogeneration‚ format. Thus, while nuclear power has historically been considered inflexible, and therefore is used only for baseload generation, by effectively using nuclear energy in cogeneration mode, it is in fact possible to make nuclear appropriately flexible.
By building a large nuclear capacity for cogeneration activities it is possible to divert the nuclear energy to provision of electrical power for the grid very cheaply when needed. This has the potential to become a very low-cost option provided the total support for the grid over the year is kept to below 20% of the total use.
However, with higher temperature Advanced Modular Reactors (AMR) this approach can be taken even further by utilising thermal energy storage, which offers a lower cost solution to energy storage. Thermal storage would be a key element for the use of nuclear heat for cogeneration and also decouples the reactor from the electricity generation process. This enables a larger generating capacity to be provided, which would in turn deliver a larger effective capacity for grid support when needed. The costs of doing this would be much lower than providing more dedicated power plants for high levels of demand but with low capacity factors. The provision of thermal storage also opens up the possibility of reactor cogeneration at industrial sites where nuclear plants would be co-located with energy-intensive industrial applications. Such sites could accept excess variable renewable electricity which would otherwise be curtailed, for applications like hydrogen production, and support them with nuclear heat to increase efficiency. This approach can also reduce carbon emissions.
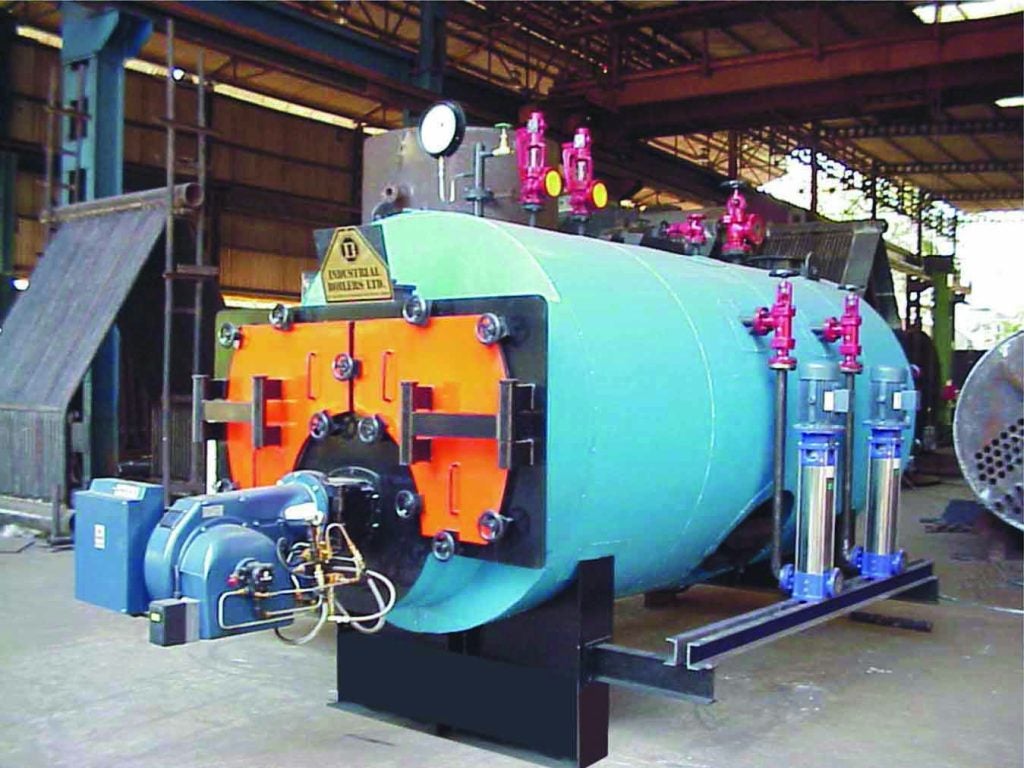
Heating up the nuclear contribution
In the “Flexible Nuclear‚ scenario outlined in the report, nuclear energy primarily delivers heat to produce hydrogen and other zero-carbon fuels, with renewables delivering the bulk of electricity generation. When renewable output drops, nuclear energy would be diverted from hydrogen and so-called e-fuels to generate electricity for the grid.
The production and use of hydrogen is increasingly being recognised as an important element of decarbonisation. As well as being a fuel itself, hydrogen is a first step in creating synthetic fuels. Hydrogen, unlike electricity or heat, can also be stored in significant amounts for long periods and can thus be part of the solution for coping with the variation in energy demand between summer and winter.
Hydrogen can be produced solely with electricity, or with electricity assisted by heat. Where heat is available at low cost, the latter option is more cost-effective, which makes the cogeneration approach especially relevant in anticipation of a future system containing a mixture of gigawatt-scale light water reactors, small modular reactors (SMRs) and AMRs, the report says. One important aspect of this is that both high-grade (>500°C) and low-grade heat (<300°C) have their place in getting more out of nuclear plants when it comes to hydrogen generation. Enabling the full potential of nuclear cogeneration will require substantial investment, but that investment can reduce CO2 emissions and reduce the overall cost of achieving net zero, whereas retaining the use of fossil fuels with CCS will not.
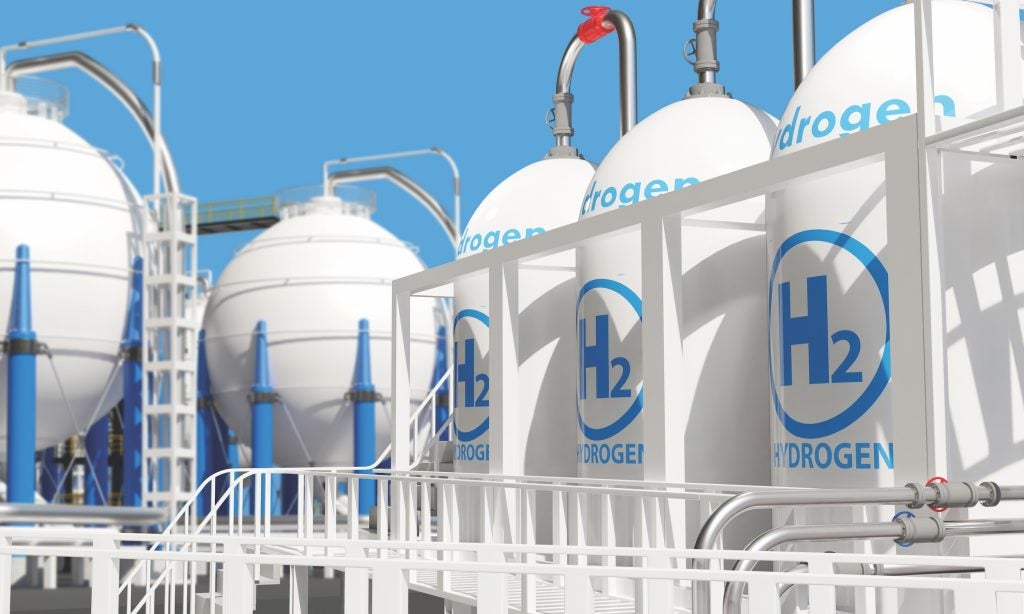
Hydrogen is currently produced largely using steam methane reformation. The CO2 produced can be reduced by CCS, but there are residual CO2 and CO2 equivalent emissions from natural gas extraction and usage. The hydrogen price from methane reforming is also dependent on the price of natural gas.
An alternative to steam reformation is to use electrolysis of water, and this is increasingly attractive as technologies for electrolysis have evolved and improved. The use of electrolysis to utilise otherwise-wasted excess renewables electricity is particularly interesting. While it is possible to produce hydrogen using electrolysis at room temperature, efficiency is vastly improved at high temperatures. Progress in high temperature Solid Oxide Electrolyser Cell (SOEC) technology renders it particularly suitable for use with heat from high temperature gas-cooled reactors (HTGRs), and it is likely that this will eventually be the cheapest route to hydrogen production. Once a low-carbon source of hydrogen is established, this can be a starting point for the production of synfuels, syngas and ammonia, which opens up a potential pathway for nuclear electricity and heat to help decarbonise several sectors where this is currently very challenging.
A further alternative for hydrogen production utilises thermochemical cycles. Various cycles have potential, and efficiencies and temperature demands vary. While studies have recognised long-term potential for thermochemical cycles, the higher Technology Readiness Level (TRL) of high temperature SOEC makes them more immediately favourable.
The potential to use heat from nuclear power stations goes further than high temperature applications for electricity generation and industry. Lower temperature heat from all nuclear technologies could be used to supply district heating for hot water and buildings. Some projects are already in progress, but their scale in the UK is very much smaller than similar projects in progress from existing reactors in other countries. This a clearly an area where efforts in pursuit of net zero should promote broader studies on what is necessary for the future, recognising both the potential for SMRs and AMRs to bring nuclear plants to many new communities and the fact that future reactors may be focused on the production of heat as well as simply electricity.
Overall, the report says, the potential for nuclear energy to contribute to economic reduction of carbon burdens is very great, and spans both the gigawatt-scale and SMR versions of LWRs, and the potential for a considerable programme of HTGRs. These possibilities combine to point to a “Flexible Nuclear‚ approach, within a grid comprising both variable renewables and nuclear, as a potential route to achieving net zero.
An economic, low-carbon solution
Increases in the lifetime cost of energy (LCOE) of various supporting power sources needed for when the renewable energy is not available is a key challenge. Many of these power sources are expensive, or limited in their availability, or have constraints on low-capacity operation. In all cases the effect of the fixed costs of the plants would cause a sharp rise in electricity costs if required to operate at low capacity factors. This would for example prevent nuclear from being competitive when used to load follow for capacity factors much below 70%. With very high renewable capacities, the use of unabated natural gas within combined cycle gas turbines or rapid response gas turbines would be expensive and difficult to provide sufficient capacity. Failure to provide this capacity would mean more investment would be required in natural gas with carbon capture and storage (CCS), hydrogen power and storage with the consequential transfer of the lower capacity factor and increased costs per unit of electricity delivered.
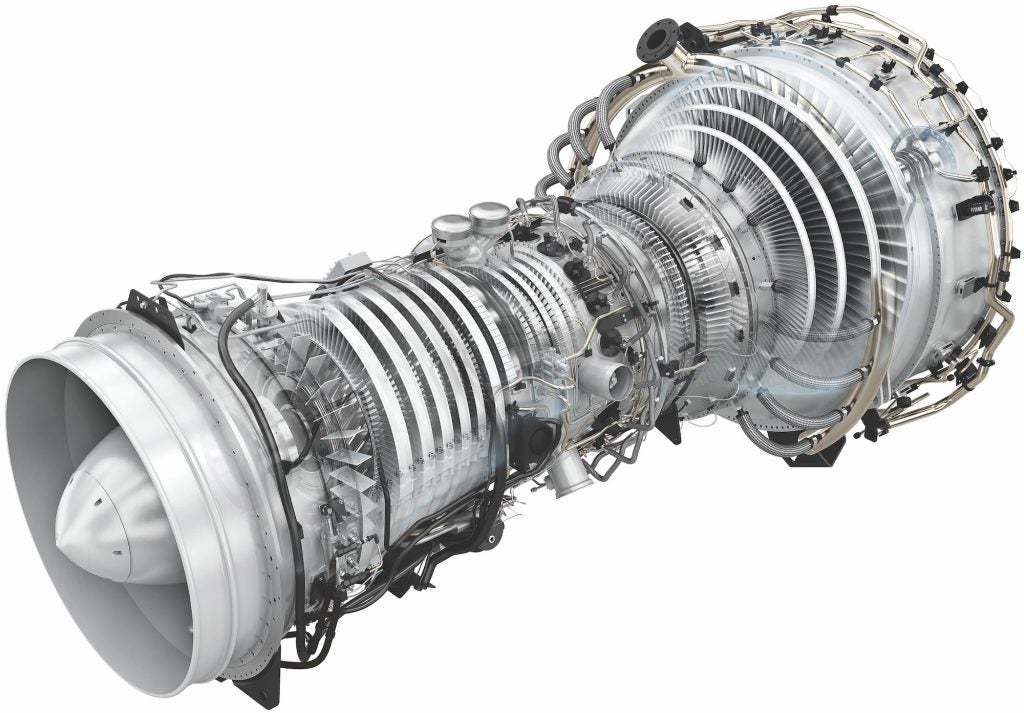
In focusing nuclear applications on cogeneration applied to hydrogen production and related low-carbon use of heat and electricity this frees nuclear to be diverted to supporting the grid when needed, thus bringing nuclear and renewables together as an entity. This is particularly true in the treatment of the potential role of HTGR reactors. It is clear that an overall solution which combines nuclear energy with renewables could provide a very low carbon solution to electricity needs, and potentially a major proportion of total energy requirements. However, while this would be made very difficult by the inflexible nature of traditional nuclear electricity generation this problem can be avoided by an approach which allows the reactor to run at its highest power and availability levels, while making economic use of the energy produced, either as heat or electricity.
Building a substantial capacity to produce nuclear hydrogen would enable part or all of that capacity to be diverted to support the grid with electricity generation when variable renewable energy output is low. This also delivers so-called “spinning capacity‚ and would mean that electricity can be quickly and easily diverted for grid support, at the expense of some hydrogen production. Reducing nuclear power for hydrogen production by, for instance 50%, would approximately double the unit cost of the hydrogen, although the fraction of the power that would need to be diverted on average over a year would be expected to be much less than 50%. It is also important to note that any diversion would occur at a time of high electricity demand and therefore higher electricity prices, so the overall economic impact on hydrogen costs would be cushioned by electricity revenues. The impact of the costs on the cogeneration activities is also small because it is taken in a region of the cost-capacity factor curve that is quite flat. This avoids the economically disadvantageous situation of nuclear plants sitting idle when renewables output is high. Conversely, dedicated peaking plants inevitably operate in a region of the cost-capacity curve that is very steep.
Building a nuclear heat market
The Dalton Institute study illustrates how considerable improvements could be made, in both net zero system economics and carbon by utilising a flexible combination of nuclear electricity and nuclear heat. This combination of nuclear electricity and heat could include both large, GW-scale reactors and Small Modular Reactors (SMRs), as well as Advanced Modular Reactors (AMRs) in particular through deployment of High Temperature Gas-cooled Reactors (HTGRs) with hydrogen generation.
However, to achieve this a number of market reforms are required and the report lays out 10 recommendations to facilitate the replacement of electricity from gas with flexible nuclear cogeneration to meet the needs of the renewable variability response.
Recommendation One
Government decision-making on the future energy mix should consider the capacity factors of new and existing infrastructure, and where these are low, seek alternatives which are potentially more cost effective.
A critical development is to recognise the opportunity to use current and advanced nuclear technologies for cogeneration rather than straightforward electricity generation. Leaning into this opportunity would enable diversion of some of the nuclear capacity as a lower cost dispatchable source of power for bridging the gap when renewables are not available and recognise the need for low-carbon hydrogen from nuclear as longer-term storage.
Recommendation Two
Since variable renewable energy generation can experience long periods with little to no output and storage options are limited in scale, government should ensure that the delivery of low-carbon, cost-effective, dispatchable electricity is prioritised to best support an effective overall system.
Recommendation Three
Government should ensure that the inefficiency of curtailment is recognised and that it is minimised as far as possible, for instance by ensuring that large-scale solar power has associated electricity storage.
One method of improving flexibility of nuclear power is to combine it with thermal storage. This arrangement of a reactor plus thermal store opens the prospect of broader commercial uptake by end users, through considerable availability of economic, flexible, useful energy output, and should be investigated.
Recommendation Four
Using reactors with thermal storage can potentially offer a cost-effective contribution to solving the problem of nuclear inflexibility. Government should prioritise research to enable an in-depth investigation of the opportunity. Nuclear energy generation must demonstrate that its economics are adequate for the roles which are proposed in the overall energy system, and its perception as being inflexible must be addressed.
Improving the economics of nuclear energy must involve reducing build times and costs by utilising fleet build and factory fabrication, and operating reactors at high capacity factors.
Recommendation Five
Establishing a holistic view of any viable “Net Zero by 2050‚ scenario the various elements must function together to produce a day-to-day and hour-to-hour regime that maintains a continuous electricity supply, optimum economics, and minimum carbon production. This has major implications on how the programme for 2050 is progressed fi combining a whole system viewpoint with an essential ability to identify, and enact, changes and programmes across the whole system is critical.
Recommendation Six
Future energy strategies should include full appreciation of effects at the whole system level, comprising generation, transmission, and storage, which must all be developed in parallel.
The level of savings that can be achieved will depend on the extent to which this flexible nuclear scenario can be implemented. This is, of course, subject to the demonstration of the economics of newer nuclear technologies fi notably fleet operation of SMRs and HTGRs. Renewable energy provides the bulk of the electricity in the Flexible Nuclear scenario but the nuclear contribution is delivered as part of cogeneration activity, and all reliance on natural gas is eliminated.
Recommendation Seven
Government assessments of the impact of new nuclear capacity should recognise and incorporate cogeneration applications (including hydrogen production). These applications ensure high capacity factors can be achieved to keep costs low and provide grid support when renewable output is low. Where appropriate, the same reasoning should be applied by the operators of existing nuclear plants.
Recommendation Eight
Success in producing hydrogen efficiently from the nuclear heat is needed, as is the presence of a robust grid, able to accommodate increased requirements and variability. This would ensure high capacity factors to further improve the economics for nuclear that embraces a fleet approach to modular new build and a beyond baseload ethos.
Recommendation Nine
Synergy is an important reality, which must be faced if an effective low-carbon energy future is to be achieved. As electricity becomes more and more the responsibility of variable renewable energy generators, lots of additional baseload nuclear generation may not just be unhelpful, but actively detrimental to the entire system, as it presents a dilemma between idling thermal generators or large-scale renewables curtailment. Intelligent planning is therefore essential, and energy decisions should not be made in isolation, but with an appreciation of how each technology fits in the whole system.
Recommendation Ten
Government planning for future nuclear deployment should envisage an integrated system where nuclear and variable renewables work in harmony through cogeneration and energy storage, while planning around energy (not just electricity) infrastructure delivery should be fully co-ordinated to best ensure a functional whole system.
Next steps on the road to a flexible nuclear and renewables future
If net zero is to be achieved, high capacities of low cost solar and wind electricity generation are needed. A standby generation of dispatchable equivalent capacity is also needed for when their output is low to ensure a reliable electricity supply. A Flexible Nuclear alternative is proposed, relying on nuclear cogeneration to provide electricity when wind and solar power are not available, and nuclear-generated electricity and heat for other low-carbon industrial applications when renewable electricity is plentiful. To achieve this, beyond specific policy recommendations, the Dalton report concludes that further research and development into thermal energy storage technology is necessary, as the technology’s engineering feasibility is central to achieving the potential economic benefits of the proposed Flexible Nuclear approach. In addition, further research into the practical constraints around delivery of the outlined scale and scope of both renewable and nuclear technologies, on the timeframes envisaged, is also needed. This would include consideration of skills (including training capability), supply chain issues, planning, community engagement, finance, and more. Given the anticipated challenges in achieving full deployment of nuclear capacity, analysis of different levels of partial Flexible Nuclear deployment would support its implementation. Furthermore, holistic modelling of integrated energy systems with links between the generation, transmission and storage of electricity, heat and hydrogen would offer important insights. A grid analysis should consider both technical and cost aspects and should look at the electricity grid, the existing gas grid, implications for hydrogen and any infrastructure needed to transmit heat from place to place in the quantities envisaged. Consideration of potential changes to markets (and how to achieve them) which might be needed to accommodate such a future energy landscape would also be of value. Currently, the market is primarily focused on providing electricity to domestic and industrial consumers. In the future, effective mechanisms to encourage and value the generation, trading and inter-conversion of electricity, heat and hydrogen will be needed.