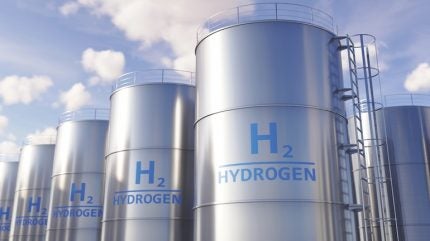
Hydrogen is seen by many as a clean energy carrier for the future. Previously it was almost entirely produced by steam reforming of fossil fuels, but it can also be produced by direct electrolysis of water. That process requires heat and electrical energy, along with a source of water, which makes it of interest to operators of nuclear and renewable energy plants, who may find themselves selling power at low or negative prices at times when generation exceeds demand. Producing hydrogen potentially offers a new market opportunity to the plant operator.
The International Atomic Energy Agency (IAEA) has published a draft report, ‘Assessing technical and economic aspects of nuclear hydrogen production for near-term deployment’ that brings together research on this topic.
Electrolysis has been used to generate hydrogen since the mid-19th century. Current technologies include Alkaline cells, which use cheap, non-noble metal catalysts for their electrodes. Meanwhile, PEM cells (proton emission membranes) can operate at high pressure, consuming less power and producing high purity hydrogen, and can be coupled directly to an intermittent source of electricity like renewables. High temperature electrolysis uses a solid oxide electrolysis cell (SOEC). It requires energy in heat and electricity and is suited for systems such as a high temperature nuclear reactor or light water reactors together with heat recovery.
One study featured, by the University of Purdue in the USA, examined the heat and operational characteristics of different types of reactor alongside the necessary characteristics for various types of hydrogen production (steam methane reforming, low temperature electrolysis, high temperature electrolysis and thermo-chemical cycles). Based on appropriate matching of temperatures, heat flux and power, the Purdue study looked at coupling systems which integrate nuclear and hydrogen production.
The report concludes that a nuclear reactor could be used to drive a steam reforming plant, a coal gasification facility, or an electrolysis plant. However, the key to coupling a nuclear reactor and thermochemical hydrogen generation (ie an electrolysis system) is an intermediate heat exchanger (IHX). In addition to providing heat to the chemical plant, the IHX acts as both an interface and an isolation device between the thermochemical plant and the nuclear reactor. The Purdue reports states that it:
- Isolates the chemical plant from the nuclear plant
- Excludes or limits radioactive contamination
- Prevents corrosive chemical reactants entering the nuclear facility
- Allows for conventional design and maintenance of the heat utilisation system.
In the event a nuclear plant is coupled with an electrolysis facility, understanding the electrolysis plant’s response to a transient event is essential to maintain nuclear safety.
In a reactor a partial loss-of-coolant accident is one of the most significant transients and could result in a severe accident. A quick shift in flow rate is a significant deviation in such a transient. Periodically, an emergency introduction of negative reactivity would be required to halt an uncontrollable nuclear reaction. The temperature of the reactor coolant’s outflow would then swiftly decrease because of the reactivity reduction. In such a situation, it would be crucial to comprehend how chemical plant reaction rates and hydrogen production rates would interact under such circumstances.
Integrating hydrogen production facilities with existing nuclear plants thus requires careful planning, design and implementation to ensure that the process is safe, efficient and economically viable. The steps and considerations for the integration of hydrogen production with existing and future nuclear plants are:
Site analysis:
- Evaluate the available space and infrastructure at the nuclear plant site to accommodate hydrogen production facilities
- Assess the availability of water, which is the primary feedstock for hydrogen production through electrolysis
- Evaluate the existing and needed infrastructure to support hydrogen production and generation
Feasibility study:
- Conduct technical and economic feasibility studies to determine the optimal method for hydrogen production to be coupled with a specific nuclear plant
- Analyse the electrical and thermal output profiles of the nuclear unit to optimize hydrogen production during periods of low electricity demand
Carry out a safety and risk assessment:
- Perform a thorough safety assessment to analyse the risks associated with integrating hydrogen production, considering hydrogen’s flammability and explosion potential
- Ensure that the integration complies with nuclear safety regulations and does not compromise the integrity of the nuclear plant
- Prepare the documentation for regulatory reviews and obtain necessary licences and permits during the design and engineering phase
Public and stakeholder engagement:
- Engage with local communities, stakeholders, and regulatory bodies to explain the benefits and safety measures of the integrated system
- Address any concerns and provide transparency throughout the process
Performance analysis:
- Continuously evaluate the performance of the hydrogen production facility to ensure it meets design specifications and production targets
- Analyse the operational data to identify areas for improving in efficiency cost-effectiveness
- Use real-world data to refine models and predictions related to maintenance, production optimisation and safety
- Consider implementing upgrades that could enhance performance or reduce costs;
- Evaluate the benefits and risks of increasing production capacity, including the potential impact on the nuclear plant and the local grid
End-of-life planning:
- Incorporate end-of-life planning for the hydrogen production facilities as part of the overall lifecycle management of the nuclear power plant
- Develop effective strategies for decommissioning the hydrogen infrastructure safely when it reaches its end of service life
As this field evolves, the successful integration of nuclear and hydrogen production will depend on careful planning, stakeholder engagement, compliance with regulatory standards and a forward-looking approach that embraces innovation and sustainability.
Producing hydrogen at a competitive price must take into account the overall costs at power-system level as well as the costs of hydrogen distribution, transport and storage.
Combining nuclear with solar to produce clean and green hydrogen
The IAEA report also includes a techno-economic study of electrolytic hydrogen production, using a nuclear-solar hybrid system (in Algeria) as an example.
Hybridisation gives flexibility and reliability to the hydrogen production system. However, the report concludes that integrating nuclear and solar in hybrid energy systems for hydrogen production is “not a trivial endeavour” because of the numerous subsystem components, complicated interconnections and interdependencies. In planning, developing and operation, the report says, the combined plant stakeholders may have conflicting goals. The IAEA analysis continues: “To guarantee the effective design, deployment and operation of the coupled system, this complexity gives rise to numerous relationships and uncertainties that need to be taken into account during system design and development stage”. It notes that existing energy infrastructure significantly influences compatibility and plant integration, and therefore the economic viability and sustainability of hydrogen production.
In a nuclear-solar PV energy system powering a low temperature electrolyser, both the nuclear and solar PV subsystems provide electricity for low temperature water electrolysis to generate hydrogen.
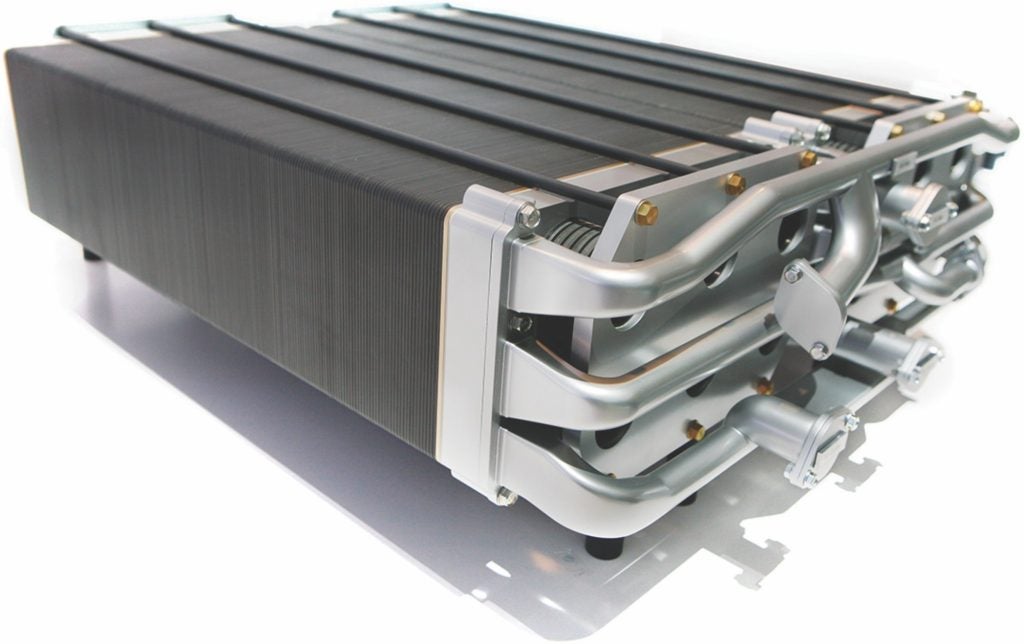
The research studied a conventional water electrolysis process powered by a hybrid solar-nuclear system consisting of a solar farm and a PWR. It found that, at low solar irradiance, the cost of hydrogen increases with an increasing solar fraction because of the low competitivity of solar in this range of solar irradiance. In contrast, at high solar irradiation, the cost of hydrogen decreases with an increasing solar fraction because the solar power is becoming more competitive than the nuclear system.
The research found that the cost of the production of the electricity of solar origin depends strongly on the values of the solar radiation incident on the PV panels. This has, of course, a direct effect on the cost of hydrogen production. At high solar irradiance (irradiance equal to or higher than 7 kWh/m2), the solar electricity is more competitive and using a large proportion of solar PV helps in bringing down the cost of hydrogen production. In this case, the solar PV subsystem saves nuclear fuel and brings down the cost of hydrogen production, while the nuclear subsystem provides the means to overcome the intermittency and the variability of solar energy.
The research compares this with the more common case where solar irradiance is not high, so the nuclear electricity is more competitive with that from solar PV. In this case, minimising the cost of hydrogen requires the contribution of solar-based electricity to be low and the solar fraction in the hybrid system should therefore be relatively small.
Similar results are obtained for PV efficiency: there is an increase in the cost of hydrogen production with an increase in the solar fraction for low efficiency PV systems and a decrease in its cost with an increase in solar fraction for high efficiency PV systems.
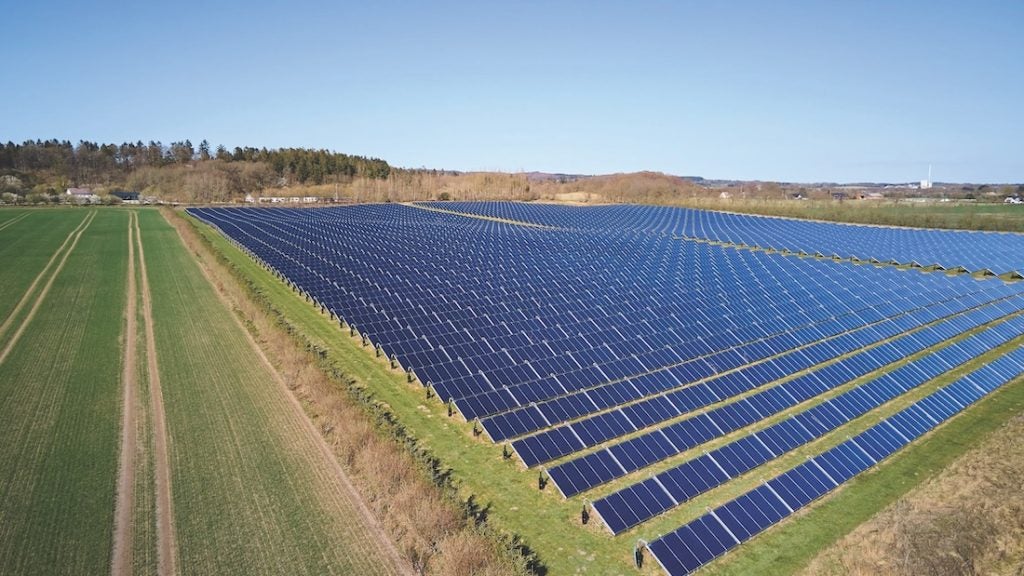
These results are of importance in the design and the operation of a hybrid nuclear solar system, for example in determining the solar fraction for optimum operation of the hybrid system that minimises the costs, helps overcome the solar intermittency and increases the time between fuel replacement operations in the nuclear reactor.
It suggests that nuclear-solar hybrid systems for hydrogen production benefit from the complementarity of the two clean energy sources: nuclear helps overcome solar intermittency, while solar helps save nuclear fuel and increases the time between reloads.