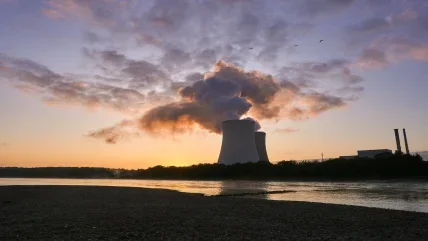
Governments are increasingly recognising that net zero solutions must be found and implemented urgently in order to meet global decarbonisation targets. Despite the impressive growth of renewables, almost all credible pathways to net zero argue this will require more than a green electricity system. Renewables and other forms of low-carbon electricity are certainly important, but decarbonising transport and heavy industry will also need low-carbon alternatives to fossil fuels. As Luis del Barrio, partner at the Madrid office of the energy & utilities practice at Arthur D. Little explains: “Electricity is not the only solution. In order to really achieve decarbonisation, we need a broader portfolio and toolkit.”
One area of growing interest is synthetic fuels or synfuels, low-carbon equivalents of conventional liquid fossil fuels. Indeed, Dr Charles Forsberg, principal research scientist at Massachusetts Institute of Technology (MIT), notes: “In the US oil products provide almost half the energy to the final consumer: residential, commercial, industrial and transportation. Electricity is less than 20% of the energy delivered to the final customer. Replace oil and decarbonise half the economy. The implied market for hydrogen and heat is larger than the electricity market.”
Typically, synfuels are compliant with international fuel standards and possess the same energy density as their fossil fuel equivalents. They can be deployed in sectors that are often considered hard to reach, notably transport. Unlike biofuels, synfuels are not necessarily reliant on organic materials to provide the molecular inputs of carbon and hydrogen. They therefore have a greater potential to scale to the larger volumes needed.
Crucially, these drop-in synfuels are able to use the same distribution infrastructure and, perhaps more importantly, existing assets like aircraft or heavy goods vehicles, without modification. Conserving existing internal combustion engines and all of the related fuel infrastructure represents both a considerable carbon saving and a substantial economic gain.
“A plane that runs with kerosene can run with synfuels without any modification. We don’t have to make any modification in almost the entire supply chain. For the production of aviation fuel we just change the oil refineries to hydrogen production plants, the same for ships and heavy duty trucks. Therefore, if we have a circular economic vision, synthetic fuel could be the most sustainable way to decarbonise the economy because we are increasing the life of existing assets and as a result, we are reducing the amount of raw materials that we are going to need,” says del Barrio.
The nuclear advantage
Given one of the key inputs for synfuels is hydrogen, low-carbon routes to hydrogen production are needed if they are to replace fossil fuels. Much of the focus of low-carbon hydrogen to date has been related to renewables such as wind and solar. However, nuclear as a low-carbon energy source is an attractive alternative and has been gaining growing interest. As del Barrio says: “To meet decarbonisation targets, nuclear synthetic fuels are going to be needed if we want to do it in an efficient and economical way. There are some countries and regions where nuclear is still not seen as a part of the decarbonisation solution, my view is it is one of the most relevant parts.”
Importantly, del Barrio highlights a number of the key benefits of nuclear-produced hydrogen in relation to that generated by renewables. He emphasises nuclear’s low cost, security of supply, reliability and flexibility. Another key advantage for nuclear over renewables is the absence of variability. This is crucial for the process side of producing hydrogen. “To achieve cheap hydrogen, we need both low-carbon electricity and electrolysers that run smoothly and for as many hours as possible. Nuclear power plants are the only technology that run 8760 hours per year with minimal downtime for maintenance,” says del Barrio.
A recent report by the Nuclear Industry Association (NIA) also highlights the characteristics of nuclear that favour its use in synfuels production. For instance, nuclear heat output can be used to provide desalination for the clean water required for hydrogen production from electrolysis. In addition, nuclear power can supply the energy needed for direct carbon capture technology and thus can create a closed carbon loop.
Given these advantages, together with its energy density and longevity, nuclear is clearly the winner when it comes to the technical aspects of producing hydrogen from low carbon sources.
It is also increasingly clear that should nuclear become established as a key source of synfuels production, the economic opportunities are substantial. According to the NIA, the global market for conventional aviation fuel alone is currently predicted to be $240b by 2026 and the global synthetic fuels market valued at $15.3bn by 2030 and $600bn by 2050. Furthermore, the NIA says, based on a survey of eight leading analyst organisations, the average prediction of the market share that synthetic fuels could achieve is over 42% of the total 2050 aviation fuel market.
Should nuclear be deployed at the scales envisaged to replace liquid fossil fuels, it represents a massive market boost for nuclear deployment. As Forsberg says: “Ultimately, liquid hydrocarbon fuels could be the largest single market for nuclear energy.”
With these kind of figures in mind the economic case for nuclear synfuel becomes compelling. “Nuclear power plants that are already completely amortised have a low cost of electricity. Running the power plant for 8760 hours with an electrolyser supports cheap hydrogen. Our analysis suggests costs below EUR2.5/kg. At this cost, synthetic fuels are competitive against other fuels like ethanol or pure kerosene. More importantly, it is much cheaper than direct electrification of transport,” del Barrio explains, referring to the process of replacing technologies like internal combustion engines that use fossil fuels with technologies like motors that use electricity directly as a source of energy. He continues: “by using nuclear to produce synthetic fuels we are able to reduce the total cost of decarbonisation for consumers. Because we are using less resources it’s also going to be more efficient, cheaper, and quicker than other options. Nuclear synthetic fuel production will be an important part of the solution for decarbonisation because the cost is going to be lower than if we have to produce synthetic fuel with renewable energy sources,” concludes del Barrio.
Barriers to nuclear deployment
Given the obvious advantages of nuclear-derived synfuels, their uptake has nonetheless been muted to date, although a number of small pilot nuclear hydrogen projects have already been launched. For example, in the US, Constellation Energy has launched a demonstration project at its Nine Mile Point Power Station in New York. Part of a $14.5m cost-shared project with the US Department of Energy (DOE), Constellation will use the hydrogen generated on-site to help cool the power plant. The DOE supported the construction and installation of a low-temperature electrolysis system at the plant that leverages the facility’s existing hydrogen storage system. The system started production in February. Similarly, in the UK EDF’s Heysham 2 plant is set for a pilot solid oxide electrolysis cell (SOEC) that will produce hydrogen for use in the production of asphalt and cement for construction materials supplier Heidelberg Materials, formerly known as Hanson. Government funding is going toward final design with a possible construction start date sometime in 2024.
Once the technology is proven it should be feasible to gain both governmental and private sector financial support. Nonetheless, despite such highlights it is evident that some barriers remain to widespread roll out of nuclear hydrogen and with it the subsequent synfuels market.
According to del Barrio, these barriers are largely not technical issues but rather policy challenges, notably public concerns that relate to safety and waste management coupled with the historic cost and construction overruns for large reactors that have also curtailed nuclear in general.
As del Barrio observes: “There is no major technical reason, rather it’s a political opinion.”
There certainly are political considerations. Post-Chornobyl and Fukushima, environmental campaigners argue that nuclear power is prohibitively dangerous as well as expensive and that plants take too long to build. Meanwhile waste management has always been contentious. Those attitudes are changing though as recognition of the looming climate catastrophe casts nuclear in a new light.
In addition, with advances in small modular reactors (SMR’s) some of the economic and timeframe arguments traditionally associated with large light water reactors become less significant.
del Barrio notes that while some issues, such as waste management, have served to impede the growth of nuclear, technical solutions are certainly possible. Further, he contrasts the remote risk of nuclear accident against the very real risk of catastrophic climate change, saying: “At the end of the day the risk of a nuclear disaster is small in comparison with the risks of a 1.5 degree increase in global average temperature.” He emphasises the relative impact on biodiversity, economic development, health and safety of a nuclear disaster in stark contrast to the impact of climate change. del Barrio all but dismisses the nuclear risks on that basis: “It’s a relatively local problem when compared with a super global problem for the population”.
But alongside the political challenges, Forsberg highlights potential economic risks for nuclear synfuels given the volatility in oil prices. “The biggest barrier for any alternative hydrocarbon liquid fuel is actually the variability in the price of oil. At $100 a barrel, some of these nuclear systems will become economic, the problem is what happens if your plant goes online when the price of crude oil is $35 a barrel?” Forsberg argues that in order secure investment in a new method of producing liquid hydrocarbon fuels some constraints must be placed on fossil fuels such as enforcing a robust carbon price to artificially increase the consumer cost of fossil fuels or alternatively a guaranteed minimum price for low-carbon equivalents.
Hydrogen needs carbon
Alongside hydrogen, the production of hydrocarbon synfuels also requires carbon. Carbon can come from various sources, including carbon capture from industrial processes or direct capture from the atmosphere. However, while direct capture raises the possibility of a circular carbon economy, del Barrio notes there are some technical issues that must be overcome: “ultimately where CO2 is derived from the air the technology is still not commercially viable. That technology is a little bit far away and quite expensive.” Instead, he points to another readily available source of carbon – biomass and waste valorisation.
“In order to make competitive synthetic fuel, we need two things, cheap CO2 and cheap hydrogen,” del Barrio explains, adding: “From biomass or biomethane production, you can have large amounts of CO2 that can be captured and that is renewable or biogenic carbon dioxide. The problem is that there is not enough biomass feed stock for all the transport sector, we need both nuclear synfuel and biofuel to complement each other.” He goes on to suggest that using the CO2 from biofuels together with nuclear-produced hydrogen could result in a symbiotic partnership with many benefits for both the industry and wider society: “We need the CO2 from biofuel production in order to produce the synthetic fuels, they are working together to achieve the cheapest solution.”
He envisages future refineries which use biomass as a raw feed stock and which together with an on-site reactor becomes a major source of low carbon liquid fuels, some of which are biogenic in origin and others which are nuclear synfuels.
While conventional biofuel production largely relies on fermentation or anaerobic digestion, cellulosic biomass – made from non-food crops and waste such as corn waste, straw or wood – has traditionally been far more challenging as a biofuel feed stock.
Forsberg says: “Currently most biofuels are from plant oils, sugar and carbohydrates because it takes less refining to convert to liquid fuels. However, feedstock is limited and conflicts with food production.” He identifies the vast quantities of cheaply available cellulosic biomass as an alternative: “It is the only feedstock option if you require massive quantities of liquid hydrocarbon fuels. There are no other choices. The first few cellulosic biomass to bio-crude oil plants are beginning operations and the most important outcome of our work is that we really can replace crude oil. Reality is slowly sinking in”.
He points to the potential role of nuclear in the use of cellulosic biomass in synfuel production and argues cellulosic biomass is a valuable and scalable source of carbon. Cellulosic biofuels can replace all crude oil but only with massive heat and hydrogen inputs. There is insufficient feed stock if biomass is used as the carbon source for the biofuels and the energy source for the conversion process and to produce the necessary hydrogen. However, he envisages gradual deployment rather than a rapid distribution. “Development of a nuclear-assisted cellulosic biofuels world will be an evolutionary development. There will be no grand opening of a new nuclear-assisted biorefinery.”
Forsberg identifies several key trends that are underway, and which plot a path for nuclear synfuel deployment in conjunction with a burgeoning biofuels sector. “Our analysis supports using existing refineries with front end changes to accept various biomass feed stocks. That is already happening. The original biofuels model was the stand-alone biofuels plant producing a final product for the customer. Most of the recent announcements are different biofuels companies producing bio-crudes shipped to local refineries. That is all driven by economics and we see acceleration of that trend.”
He also mentions the attractive heat characteristics of nuclear power which complement the massive heat demands of chemical plants and refineries. Forsberg highlights the recent announcement by DOW Chemical which intends to install four high-temperature SMRs at the Seadrift chemical site in Texas. Featuring X-energy’s Xe-100 SMR design, DOW’s project aims to deliver power and steam production to match the sites requirements with zero carbon emissions. Each reactor will produce 200 MW of heat, collectively delivering 800 MW of heat. DOW estimate a 400,000 tonne reduction of CO2 emissions per year. Construction is expected to begin in 2026 and be completed by the end of this decade. Forsberg comments: “What is noteworthy is that these reactors are designed for the chemical market that includes refineries”. The heat inputs to the chemical and biofuels industries may exceed the existing heat outputs of the current nuclear fleet. The near-term hydrogen source will likely be natural gas to hydrogen with sequestering carbon dioxide where there is cheap natural gas and good sequestration sites. Nuclear is the hydrogen everywhere choice.
The future beckons
Despite the political and economic challenges, del Barrio is emphatic that the nuclear resurgence is significant for a future synfuels market.
“When you take the lifecycle costs you will see that synthetic fuels running on nuclear power plants are the cheapest option and are needed to achieve the total decarbonisation of the economy,” he continues, “as nations start building reactors and getting these new machines online, that will then lead to synthetic fuel production, then we need to use that tool to decarbonise our liquid fuels transport system”.
del Barrio concludes: “There are countries that are already pushing for nuclear power and when they have nuclear power, they will have nuclear synthetic fuels.”
Asked about the global situation Dr Forsberg sums up: “The way I would put it is that we’re stumbling around in the dark if you look at a lot of carbon policies. They’re based more on what we wish to be true rather than what is true. The engineering realities at the end of the day, like available forms of carbon are going to drive things.”
Dr Forsberg also highlights the role of nuclear in meeting the need for low-carbon energy: “You need steady-state heat for the chemical industry which matches nuclear. In a low-carbon world, your options are limited, you either burn-natural gas with carbon capture or use nuclear. You have an industry that intrinsically needs an awful lot of heat, which of course nuclear reactors produce.”
Looking ahead del Barrio is also optimistic: “In the fifties we were dreaming of having nuclear cars and I think in the future we are going to run vehicles on nuclear, because we will have synthetic fuels produced with nuclear fuels. That’s going to be a reality. Whether they run on electricity that is coming from nuclear power plants, whether they’re running on fuel that is coming from nuclear power plants, at the end of the day, nuclear is going to be a part of the solution.”
Author: Tim Nadin